BioByte 100: integrating biological complexity into AI, scaling connectomics to the human brain, deep brain stimulation for spinal cord injury, ESM Cambrian launch
Welcome to Decoding Bio’s BioByte: each week our writing collective highlight notable news—from the latest scientific papers to the latest funding rounds—and everything in between. All in one place.
What we read
Blogs
“Wearable” devices for cells [Adam Zewe, MIT News, October 2024]
Researchers at the MIT Media Lab have developed subcellular-sized wearable devices that can fit individual neurons. By shining light on thin films of an azobenzene polymer, Sarkar et al. were able to demonstrate the ability of the polymeric sheets to curl around axons of animal subjects in vitro. One of the primary challenges with designing such interfaces is that they must not damage the cell by curling too tightly and yet remain highly conformable, lest they be unable to make intimate contact with the axon. By controlling the polarization and duration of exposure, the researchers were able to dictate the degree of curling and, by doing so, demonstrate that azobenzene exhibited the optimal properties to achieve this aim of axonal contact without inducing cellular damage. When combined with its established cytocompatibility, this result indicates great promise for translation to in vivo studies.
The films themselves were fabricated with a stamp. Sarkar et al. placed a drop of the liquid polymer onto a water-soluble substrate, then pressed their stamp onto the droplet, forming the desired shapes. To isolate the polymers and suspend them in solution, the researchers etched away the remaining exposed substrate, subsequently placing the devices in water. In doing so, the substrate dissolves in the water, leaving the devices suspended within the solution. The researchers propose that this method of preparation makes the devices highly compatible with injection.
These azobenzene polymers have a number of potential applications as single-cell wearables due to their unique properties. Because azobenzene polymers act as insulators, these devices could be used as a replacement for myelin, which would be particularly relevant for the treatment of diseases such as multiple sclerosis where myelin sheets are irreversibly lost. Additionally, the researchers illustrated the propensity of the devices for use in tandem with optoelectrical elements, creating single cell circuits that could have significant utilization for stimulating or monitoring subcortical activity.
Although promising, there is still much work to bring about these polymers. For one, they have thus far only been synthesized in a cleanroom. In order to scale the devices, this manufacturing barrier must ideally be surmounted. Furthermore, greater exploration of surface modifications enabling discrimination and localization of the polymer devices to certain target cells or regions in the brain is necessary.
Fluorescent Tags Are Basically Never Silent [Derek Lowe, Dec 2024]
The latest blog from Derek Lowe discusses the "Tagger's Paradox" in chemical biology, which mirrors the observer's paradox in quantum mechanics and social sciences. The core issue is that fluorescent tagging, while a workhouse for understanding biology and essential for observing biomolecules in cells, can significantly alter the very behaviors being studied. Fluorescent tags allow researchers to track proteins and other biomolecules by making them emit light of specific wavelengths, enabling visualization of their location, movement, and interactions. However, these tags can affect crucial properties like membrane transport, binding mechanisms, stability, and various downstream processes, making it impossible to assume that labeled molecules behave identically to their unlabeled counterparts.
The blog discusses a recent preprint detailing a specific case study examining the effects of fluorescent tagging on biomolecular condensates, specifically focusing on the yeast protein Dhh1 in P bodies (RNA-processing condensates). The researchers found that various fluorescent tags (GFP, mKate2, mVenus, mCherry, mNeonGreen, mRuby2) and His tags significantly altered the protein's behavior both in vitro and in cells. These modifications affected critical parameters including condensation threshold concentrations, RNA sensitivity, pH responsiveness, expression levels, and P-body formation patterns. Notably, while adding small amounts of labeled proteins to unlabeled systems helped mitigate some effects in vitro, this approach proved less effective in cellular experiments. The study revealed that N-terminal tags generally caused fewer disruptions than C-terminal tags for Dhh1, though this specificity would need to be verified for other proteins of interest.
These findings underscore a fundamental challenge in modern biology: our primary tools for observing molecular processes may be systematically skewing our understanding of cellular mechanisms, requiring careful validation and potentially new approaches for studying biomolecular behaviors.
A roadmap to scale connectomics to entire mammalian brains [E11 Bio, December 2024]
E11 Bio introduces PRISM, a new platform to make brain connectivity mapping (connectomics) more efficient, affordable, and scalable. This technology aims to transition from mapping small brains, like the recently completed fly connectome, to larger, more complex models, such as the mouse brain, which is closer to human brain structure. PRISM addresses key challenges in scaling connectomics, particularly the cost and time associated with human proofreading, which accounts for over 95% of current costs.
PRISM combines three innovations to tackle these barriers: protein cell barcoding, expansion microscopy, and self-proofreading AI segmentation. Protein barcoding assigns each neuron a unique molecular ID, simplifying differentiation under light microscopy. Expansion microscopy enhances imaging resolution while reducing costs, and advanced AI models analyze barcoded images, minimizing the need for manual error correction. Together, these advancements could reduce the cost of connectomics by 100-fold, making large-scale brain mapping feasible.
Beyond reducing costs, PRISM supports key scientific goals: creating blueprints for biologically inspired AI, understanding abnormal brain connectivity in diseases, and exploring how memories and traits like creativity are encoded in brain circuits. E11 Bio plans to release a detailed preprint and data from a pilot mouse hippocampus study in early 2025, paving the way for broader adoption and further development of PRISM. As a non-profit, E11 Bio is committed to sharing its methods and findings to advance connectomics research globally.
Papers
Hypothalmic deep brain stimulation augments walking after spinal cord injury [Courtine et al., Nature Medicine, December 2024]
A groundbreaking study published in Nature Medicine has revealed a promising new approach to improving walking ability after spinal cord injury (SCI) through deep brain stimulation (DBS) of the lateral hypothalamus. The research team made their initial discovery by using advanced brain imaging techniques, which identified the lateral hypothalamus (LH) as a crucial region involved in walking recovery after SCI. Specifically, they found that glutamatergic neurons in this brain area play a vital role in walking function.
The researchers first tested their findings in animal models, where they demonstrated that stimulating LH glutamatergic neurons in mice and rats with SCI led to immediate improvements in walking ability. These neurons were found to work through connections with the ventral gigantocellular nucleus to influence walking function. Both electrical stimulation through DBS and genetic stimulation techniques proved effective in animal studies.
Building on these promising results, the team conducted a pilot clinical trial with two participants who had chronic incomplete SCI. The researchers surgically implanted DBS electrodes in the participants' LH regions and observed remarkable outcomes. When the stimulation was turned on, both participants showed immediate improvements in their walking ability. After undergoing three months of rehabilitation combined with DBS, they demonstrated lasting improvements that persisted even when the stimulation was turned off. Following the study, one participant gained the ability to walk without orthoses, while the other became capable of climbing stairs independently—their rehab goals prior to treatment.
This study represents a significant advancement in the field of neuromodulation therapy for SCI. It's the first demonstration that LH stimulation can improve walking after spinal cord injury, offering both immediate benefits during stimulation and long-term recovery when combined with rehabilitation. The findings open a new therapeutic avenue for treating SCI patients and demonstrate how targeting specific brain regions can help restore lost neurological functions after injury.
Bio-inspired AI: Integrating Biological Complexity into Artificial Intelligence [Nima Dehghani et al., aRxiv, Nov 2024]
Deep learning, recurrent networks and neuromorphic computing are all loosely based on neuroscientific concepts. Transformers mimic the interconnectivity found within astrocyte networks in the brain and convolutional neural networks mimic the visual cortex through its detection in early layers of simple features such as edges and contrasts and in deeper layers then integrating these into complex patterns and objects.
The authors argue, however, that in order to truly mimic the complexity of biological intelligence we must use concepts beyond neural networks. It is clear that intelligence is found in life without spiking neurons: “slime molds like Physarum polycephalum can find the shortest path through a maze to reach food sources, effectively solving complex optimization problems without any neural structures. Plants exhibit sophisticated behaviors such as opening and closing stomata in response to environmental conditions and adjusting growth patterns toward light sources through hormonal signaling pathway”
How can we use biology to further advance AI?
Contextual information processing: biological intelligence relies heavily on context, nonlocality, and adaptive feedback. Unlike deterministic algorithms, biological systems interpret information based on current context, with meaningful information emerging from interactions across multiple scales within a hierarchical architecture. This mirrors processes like gene expression in cells, where DNA functions as a dynamic backbone influenced by the cellular environment rather than a rigid blueprint
Trial and error as a fundamental strategy: In complex environments, organisms cannot rely on precomputed optimal solutions. This approach enables effective exploration without exhaustive search, favoring adaptability over perfection. Ashby demonstrated that parallel trial-and-error strategies can rapidly solve high-dimensional problems infeasible for serial exhaustive methods.
Maintaining stability through hierarchical organization: Biological systems maintain stability through modular organization - subsystems performing specific functions. This modular and hierarchical organization enables flexibility, allowing systems to adapt without becoming unstable.
Hierarchy and information abstraction: Hierarchy involves organizing nested components for information abstraction and efficient processing, facilitating renormalization processes that summarize detailed lower-level information at higher levels. This helps the handling of complexity effectively.
Beyond deterministic algorithms: “Bayesian inference, relying on hierarchical generative models and prior assumptions, cannot achieve the contextual, non-optimal generality of biological intelligence due to its reliance on predefined priors, which limits exploration and adaptability.”
Physical computing and compositionality: by focusing on how components compose and interact in physical computing, systems that engage with complex dynamics can be created, akin to biological systems
Tracking antigen-specific T cell response to cancer immunotherapy [Shagina et al., bioRxiv, 2024]
In this paper, the authors developed a systematic method to identify and track T cells that specifically target B16 melanoma antigens in mice. Using a vaccination-based approach combined with advanced sequencing techniques, they identified 59 distinct TCR β CDR3 motifs and 485 TCR β CDR3 amino acid variants that recognize 14 different melanoma peptides, and generated a public database (available through VDJdb) to support future immunotherapy research.
They vaccinated C57Bl/6-FoxP3EGFP mice with melanoma peptides in their footpads, and followed this with collection and sorting of T cell subsets from draining lymph nodes. The team used 5'RACE with unique molecular identifiers for TCR sequencing and used computational analysis to identify TCR patterns specific to each peptide. Their pipeline successfully identified TCR clusters that were consistently enriched in response to particular peptides compared to others.
To validate their findings, the authors applied their method to study immune responses in mice treated with anti-CTLA4 immunotherapy for B16F0 melanoma. They discovered that tumors promote the development of regulatory T cells (Tregs) carrying tumor-specific TCRs similar to those found in helper T cells. Notably, while these TCR patterns were originally identified in helper T cells and absent in Tregs during vaccination, they appeared prominently in Tregs of tumor-bearing mice.
Their data revealed that CTLA4 blockade led to increased clonality in helper T cells but not in Tregs, indicating therapy-induced expansion of specific T cell clones. The authors also found that certain peptides, including p5, p20, p25, p30, and p50, consistently showed stronger immune responses across different lymph nodes and mouse groups, suggesting higher immunogenicity. This research provides a universal method for studying mouse T cell responses at the antigen-specific level, and represents a powerful tool for evaluating cancer immunotherapies preclinically.
Notable deals
AI Proteins has entered into a collaboration and option agreement with Bristol Myers Squibb (BMS) to design and optimize miniproteins targeting a series of BMS-nominated targets. The deal, which has a total potential value of $400 million, aims to harness the unique properties of miniproteins—small, rigid scaffolds that can be produced recombinantly or synthetically. While their compact size often results in a shorter serum half-life, miniproteins are capable of high-affinity binding and can access tissues and compartments that are often inaccessible to larger protein therapeutics. This collaboration represents a significant step in leveraging miniproteins to address unmet needs in drug discovery and development.
Oxford Nanopore and UK Biobank are partnering to create the world’s first large-scale epigenetic dataset, mapping 50,000 samples to advance early detection, diagnosis, and treatment of diseases like cancer and dementia. Using Oxford Nanopore’s sequencing technology, which captures 98% of epigenetic markers, the initiative will provide unprecedented insights into how lifestyle and environmental factors influence disease.
Pfizer has expanded its collaboration with Flagship Pioneering startups, enlisting Ampersand Biomedicines and Montai Therapeutics to develop novel treatments for obesity and lung cancer, respectively. Ampersand, leveraging its computational platform to target specific tissues and minimize side effects, will focus on creating localized obesity treatments. Montai, using AI-driven analysis of “Anthromolecules” derived from natural sources, aims to discover small molecules for lung cancer therapies, aligning with Pfizer’s oncology priorities following its Seagen acquisition.
What we liked on socials channels
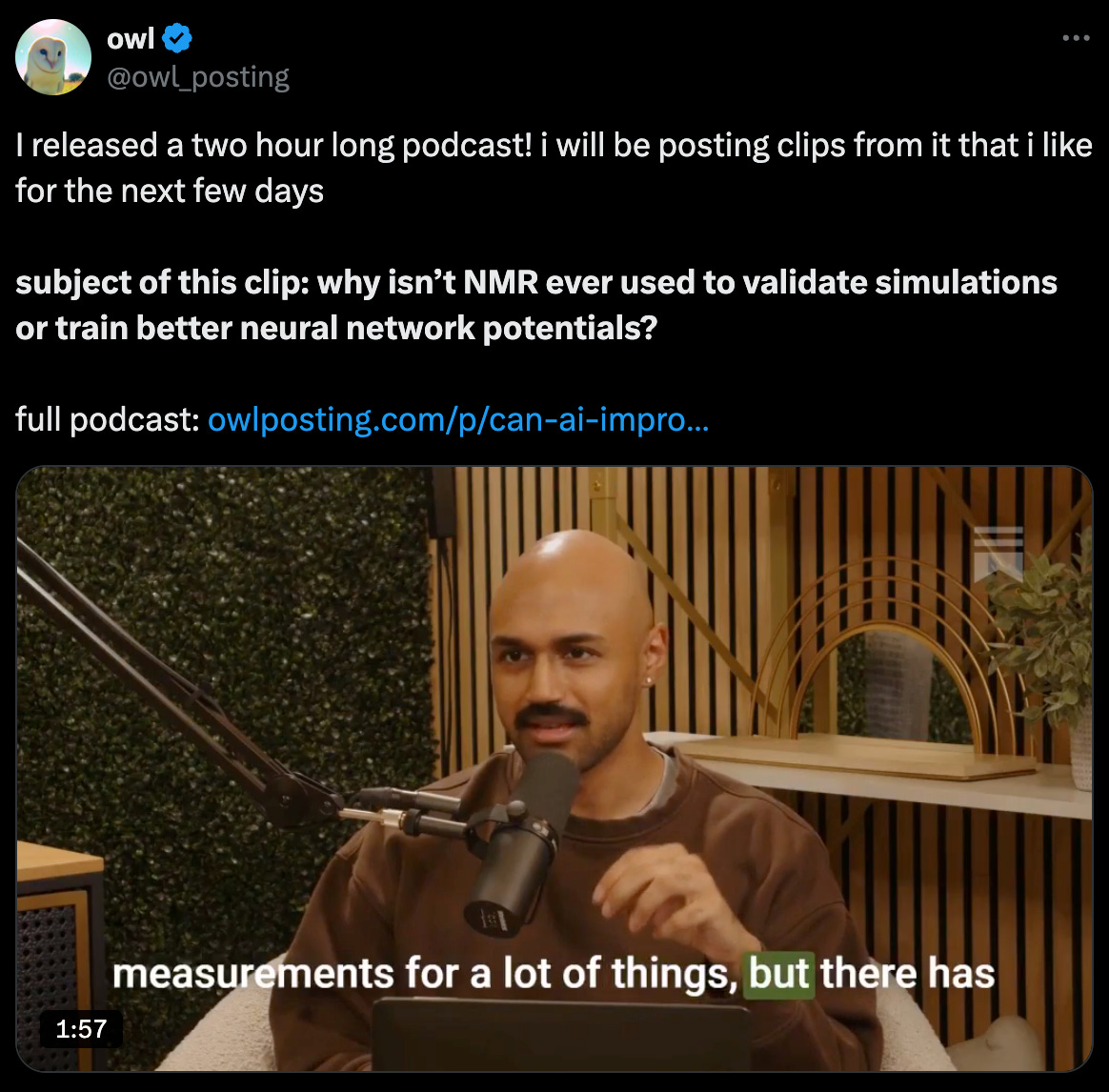
In case you missed it
ESM Cambrian: Revealing the mysteries of proteins with unsupervised learning
Field Trip
Did we miss anything? Would you like to contribute to Decoding Bio by writing a guest post? Drop us a note here or chat with us on Twitter: @ameekapadia @ketanyerneni @morgancheatham @pablolubroth @patricksmalone